Introduction: From the initial report of intraoperative radiofrequency (RF) ablation causing esophageal injury,GIL01 atrioesophageal fistulas (AEF) have been reported in percutaneous atrial fibrillation RF ablations.SCA04,PAP04 Atrioesophageal fistulas have been estimated to occur in as many as 1% of AF ablationsDOL03 but a likely accepted event rate is less than 0.1%.PAP04,SCA04,CUM06 The mortality associated with AEF is devastating and was found to approach 100% in the largest published registry of AEF.CUM06 This is in stark contrast to a near zero death rate of atrial perforations during RF ablation.BUN05 An article by Müller et al (http://www.heartrhythmjournal.com/article/S1547-5271(15)00418-X/abstract) examined the high incidence of esophageal lesions after atrial fibrillation ablations related to the use of esophageal temperature probes. Multivariate analysis revealed the use of the temperature probe was the only independent predictor of esophageal lesions. Finally, data in Heart Rhythm examined the rate of atrioesophageal fistula formation with contact force (CF) sensing catheters versus non-CF-sensing catheters. Black-Maier et al found the “occurrence of atrioesophageal fistula formation accounted for a 5-fold higher proportion of all MAUDE medical device reports of injury or death with CF-sensing catheters compared with non-CF-sensing catheters.”
Value of Imaging: The value of intracardiac imaging via radial intracardiac echo (ICE) cannot be underestimated given the nonuniform thickness and variable course along the posterior wall of the left atrium.SAN05,GOO05,REN06 The proximity of the esophagus to the left pulmonary venous antrum is depicted in Figure 1. Typically, patients with AEF present a mean of 12.3days after their procedure;CUM06 however, presentation within 3-5days of the ablation has been reported.PAP04 Findings on CT scans can be non-specific but, infected pleural and pericardial effusions may suggest esophageal contamination of the pleural spaces. CT scan of the chest (without oral contrast) with the presence of intravenous contrast seen in the esophagus or surrounding posterior mediastinum would imply a fistulous connection.MAL07 Additionally, one may note a narrowed, irregular, and ulcerated pulmonary vein, posterior left atrial wall thickening, posterior mediastinal fat induration, or pneumomediastinum.MAL07
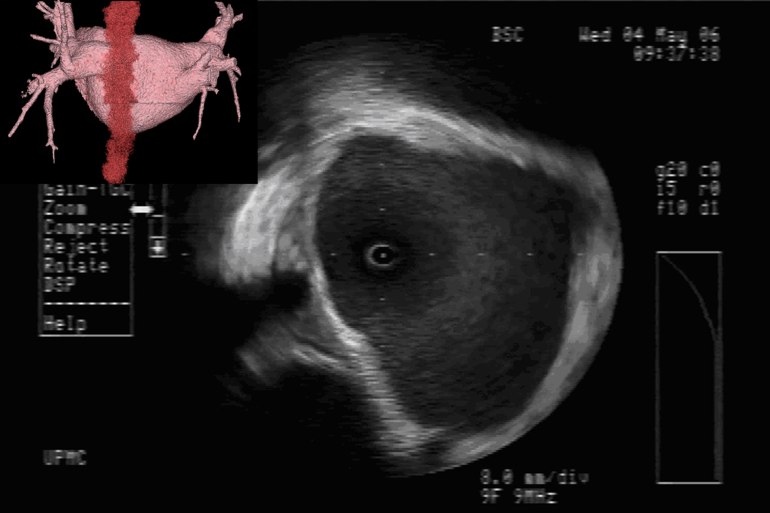
Mechanism of Esophageal Injury: Finite-element analysis supports that esophageal injury is exclusively due to thermal conduction from the atrium.BER05 Esophageal injury can occur despite small electrode size, low power (<30W), and low electrode temperature (34°C). There are two caveats however, irrigated electrodes and electrode-endocardial contact verification (direct visualization with ICE or force-sensing) may increase power delivery to the tissue.
Avoiding Esophageal Injury: There has been much enthusiasm to determine means by which esophageal injury can be avoided. These include echocardiographic monitoring for microbubble formation,CUM05 the use of cryoablation to lower esophageal ulceration,RIP07 plan ablations to avoid esophagus by creating virtual esophageal tube using electroanatomic mapping,SHE07 esophageal irrigation to lower esophageal temperature,TSU07 and physically deflecting the esophagus away from the ablation site.HER06 The study by Muller et alMUL15 suggests the possibility that esophageal temperature probes may increase susceptibility to esophageal lesions. Figure 2 shows ICE images before and after orogastric tube removal. One notes the signature of the OGT at 8 o’clock in the left image. There is a small indentation in the posterior left atrial wall at the site of the OGT. On the right, imaging demonstrates this indentation is resolved after removal of the OGT. I will often remove esophageal instrumentation to avoid any possible displacement of the esophagus towards the left atrium.
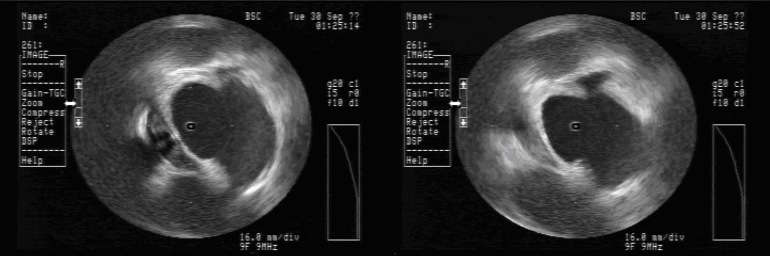
CF-sensing catheters have certainly enhanced ability to deliver more consistent lesions however, there are clearly limitations when the operator cannot see real-time electrode-endocardial contact. There have been many times where I have seen left and right atrial tenting due to catheter contact at less than 10g of force. Force sensing has certainly added to our armamentarium but I would caution all that there is more to ablation than contact force.
Note: These radial ICE images would not be possible without my mentor, Dr. David Schwartzman (Pittsburgh, PA).
REFERENCES:
GIL01 Gillinov AM, Pettersson G, Rice TW, “Esophageal injury during radiofrequency ablation for atrial fibrillation,” J Thor Card Surg, V. 122, No. 6 (December 2001), pp. 1239-1240.
SCA04 Scanavacca MI, D’Avila A, Parga J, Sosa E, “Left Atrial-Esophageal Fistula Following Radiofrequency Catheter Ablation of Atrial Fibrillation,” J Cardiovasc Electrophysiol, V. 15, No. 8 (August 2004), pp. 960-962.
PAP04 Pappone C, Oral H, Santinella V, Vicedomini G, Lang CC, Manguso F, Torracca L, Benussi S, Alfieri O, Hong R, Lau W, Hirata K, Shikuma N, Hall B, Morady F, “Atrio-Esophageal Fistula as a Complication of Percutaneous Transcatheter Ablation of Atrial Fibrillation,” Circulation, V. 109 (June 8, 2004), pp. 2724-2726.
DOL03 Doll N, Borger MA, Fabricius A, Stephan S, Gummert J, Mohr FW, Hauss J, Kottkamp H, Hindricks G, “Esophageal perforation during left atrial radiofrequency ablation: Is the risk too high?” J Thor Cardiovasc Surg, V. 125, No. 4 (April 2003), pp. 836-842.
CUM06 Cummings JE, Schweikert RA, Saliba WI, Burkhardt D, Kilikaslan F, Saad E, Natale A, “Brief Communication: Atrial-Esophageal Fistulas after Radiofrequency Ablation,” Ann Int Med, V. 144, No. 8 (18 April 2006), pp. 572-574.
BUN05 Bunch TJ, Asirvatham SJ, Friedman PA, Monahan KH, Munger TM, Rea RF, Sinak LJ, Packer DL, “Outcomes After Cardiac Perforation During Radiofrequency Ablation of the Atrium,” J Cardiovasc Electrophysiol, V. 16, No. 11 (November 2005), pp. 1172-1179.
SCH06 Schwartzman DS, Nosbisch J, and Housel Debra, “Echocardiographically guided left atrial ablation: Characterization of a new technique,” Heart Rhythm, V. 3, No. 8 (August 2006), pp. 930 –938.
SAN05 Sanchez-Quintana D, Cabrera JA, Climent V, Farre J, de Mendonca MC, Ho SY, “Anatomic Relations Between the Esophagus and Left Atrium and Relevance for Ablation of Atrial Fibrillation,” Circulation, V. 112 (September 6, 2005), pp. 1400-1405.
GOO05 Good E, Oral H, Lemola K, Han J, Tamirisa K, Igic P, Elmouchi D, Tschopp D, Reich S, Chugh A, Bogun F, Pelosi F Jr, Morady F, “Movement of the Esophagus During Left Atrial Catheter Ablation for Atrial Fibrillation,” JACC, V. 46, No. 11 (December 6, 2005), pp. 2107-21190.
REN06 Ren J-F, Lin D, Marchlinski FE, Callans DJ, Patel V, “Esophageal imaging and strategies for avoiding injury during left atrial ablation for atrial fibrillation,” Heart Rhythm, V. 3, No. 10 (October 2006), pp. 1156-1161.
MAL07 Malamis AP, Kirshenbaum KJ, and Nadimpalli S, “CT Radiographic Findings: Atrio-esophageal Fistula After Transcatheter Percutaneous Ablation of Atrial Fibrillation,” J Thorac Imaging, V. 22, No. 2 (May 2007), pp. 188-191.
BER05 Berjano EJ and Hornero F, “What affects esophageal injury during radiofrequency ablation of the left atrium? An engineering study based on finite-element analysis,” Physiol Meas, V. 26 (2005), pp. 837-848.
CUM05 Cummings JE, Schweikert RA, Saliba WI, Burkhardt JD, Brachmann J, Gunther J, Schibgilla V, Verma A, Dery MA, Drago JL, Kilicaslan F, Natale A, “Assessment of Temperature, Proximity, and Course of the Esophagus During Radiofrequency Ablation Within the Left Atrium,” Circulation, V. 112 (July 26, 2005), pp. 459-464.
RIP07 Ripley KL, Gage AA, Olsen DB, Van Vleet JF, Lau C-P, Tse H-F, “Time Course of Esophageal Lesions After Catheter Ablation with Cryothermal and Radiofrequency Ablation: Implication for Atrio-Esophageal Fistula Formation After Catheter Ablation for Atrial Fibrillation,” J Cardiovasc Electrophysiol, V. 18, No. 6 (June 2006), pp. 642-646.
SHE07 Sherzer AI, Feigenblum DY, Kulkarni S, Pina JW, Casey JL, Salka KA, Simons GR, “Continuous Nonfluoroscopic Localization of the Esophagus During Radiofrequency Catheter Ablation of Atrial Fibrillation,” J Cardiovasc Electrophysiol, V. 18, No. 2 (February 2007), pp. 157-160.
TSU07 Tsuchiya T, Ashikaga K, Nakagawa S, Hayashida K, Kugimiya H, “Atrial Fibrillation Ablation with Esophageal Cooling with a Cooled Water-Irrigated Intraesophageal Balloon: A Pilot Study,” J Cardiovasc Electrophysiol, V. 18, No. 2 (February 2007), pp. 145-150.
HER06 Herweg B, Johnson N, Postler G, Curtis AB, Barold SS, Ilercil A, “Mechanical Esophageal Deflection During Ablation of Atrial Fibrillation,” PACE, V. 29 (September 2006), pp. 957-961.
MUL15 Müller P, Dietrich J-W, Halbfass P, Abouarab A, Fochler F, Szöllösi A, Nentwich K, Roos M, Krug J, Schade A, Mügge A, Deneke T, “Higher incidence of esophageal lesions after AF ablation related to the use of esophageal temperature probes,” Heart Rhythm, Published Online: April 03, 2015.